Good News — Bad News: Combined Ocean Change Drivers Decrease Survival but Have No Negative Impact on Nutritional Value and Organoleptic Quality of the Northern Shrimp
- 1Département Biologie, Chimie, Géographie, Université du Québec à Rimouski, Rimouski, QC, Canada
- 2Institut des Sciences de la mer, Université du Québec à Rimouski, Rimouski, QC, Canada
- 3Institut Maurice-Lamontagne, Fisheries and Oceans Canada, Mont-Joli, QC, Canada
- 4Merinov, Gaspé, QC, Canada
Nutritional and organoleptic qualities (taste, smell, texture, appearance) are key characteristics of seafood when it comes to defining consumer choices. These qualities, which are determined by the biochemical properties of the seafood, can be altered by environmental conditions, such as those imposed by ongoing global ocean change. However, these effects have rarely been studied despite their potential important economic and dietary implications: many human communities depend upon seafood as a primary source of nutrition and/or income from the associated seafood industry. The Northern shrimp, Pandalus borealis, makes the 3rd most valuable fishery in Eastern Canada, and figures among the most important fisheries in the North-Eastern Atlantic. This study aimed to determine the impact of combined ocean warming, acidification and hypoxia on (a) muscle mineral content as proxy for nutritional quality, and (b) the taste, smell, texture, and appearance as proxies for organoleptic quality of this commercially important species. These proxies were determined after an exposure of 30 days under laboratory conditions to different ocean global change scenarios of temperature (2, 6, and 10°C), pH (7.75 and 7.4) and oxygen (100 and 35% relative to air saturation), in isolation and in combination. Shrimp survival was significantly lower (68%) for shrimp exposed to warming and low pH, and even lower (37%) when hypoxia was superimposed, compared to an average survival of 88% for all other treatments. Mineral contents were globally higher in shrimp exposed to the highest temperature, while organoleptic attributes were comparable across all scenarios tested. Thus, while we do not expect nutritional value and organoleptic quality of shrimp, broadly speaking, to be altered by global changes even in areas where conditions will correspond to our warmest (10°C) and lowest pH (7.4) scenarios, the lower survival rate we report could negatively impact the viability of shrimp populations and consequently the shrimp industry. This may be particularly true for areas that are currently becoming or are expected to become hypoxic.
Introduction
Seafood is a fundamental component in human nutrition, along with local and global economies. It provides a valuable source of proteins, lipids, vitamins and essential minerals (Venugopal and Gopakumar, 2017). Between 1961 and 2016, global human consumption of seafood has annually increased by 3.2%, while in 2015 alone, about 17% of animal protein consumed worldwide came from seafood (FAO, 2018). With the continuous demographic growth of the human population, the consumption of seafood will become increasingly more important, helping guaranteeing global food security (Tacon and Metian, 2013). According to FAO predictions, fishery production will reach 201 M tons in 2030, which represents an 18% increase compared to 2016 (FAO, 2018).
Marine organisms are facing the simultaneous change of a number of environmental parameters, including increasing temperature and pCO2 levels, and decreasing pH and oxygen (O2) levels as a consequence of global changes (GC) (Caldeira and Wickett, 2003; Sabine et al., 2004; Gruber, 2011). Among various ocean global change drivers, ongoing ocean warming (OW) and ocean acidification (OA), alone or combined, have been shown to negatively impact the survival, development and physiology of several marine invertebrates in the future ocean, including shellfish (e.g., Small et al., 2015; Pillet et al., 2016; Arnberg et al., 2018; Dworjanyn and Byrne, 2018).
Recent advances in environmental physiology have shown that ocean changes can also alter biochemical properties of seafood, including proximate composition: i.e., proteins (Tate et al., 2017), lipids (Anacleto et al., 2014; Ab Lah et al., 2017; Tate et al., 2017), and vitamin and mineral contents (López et al., 2010). These changes in biochemical properties can affect nutritive value, and possibly palatability and organoleptic quality (i.e., taste, smell, texture, appearance) of harvested species supporting important fisheries and coastal community economy. This being said, to date, only few studies have shown the impact of single environmental drivers on organoleptic quality of shellfish (Papadopoulos and Finne, 1986; Dupont et al., 2014; Lemasson et al., 2017); with negative impacts being reported in only one case (Dupont et al., 2014). To our knowledge, no study has so far explored the potential impacts of combined GC drivers on seafood quality. Characterizing the nutritional and organoleptic properties of shellfish species within the context of ongoing GC is necessary to better evaluate putative changes in food security and the fate of marketed products (Hilmi et al., 2013).
The Northern shrimp Pandalus borealis (Krøyer, 1838) is among the three most lucrative harvested species in Canada, with total Atlantic landings valued at 315.68 M$ in 2017 (DFO, 2018a, including landings from Nova Scotia, New Brunswick, Québec and Newfoundland). Northern shrimp (“shrimp” hereafter) fisheries generate significant economic, social and nutritional benefits for numerous coastal allochthonous and autochthonous communities (MAPAQ, 2015). Crucially, all shrimp populations exploited in Eastern Canada and North-Eastern United States (i.e., Maine) have been declining at a tremendous rate in recent years, driven by environmental changes which can be region-specific: including increasing temperatures, decreasing pH and O2 and an increase in predator pressures (Hunter et al., 2007; DFO, 2018b; Bourdages and Marquis, 2019).
Most of the shrimp populations in Eastern Canada live in deep, cold benthic environments (100–600 m – DFO, 2016) at 1–6°C (Orr and Sullivan, 2013; Hardie et al., 2015; Siferd, 2015; DFO, 2016). These populations may face a 4°C increase by 2100, bringing them to approx. 6°C (RCP 8.5, IPCC, 2014). However, the shrimp population of the St. Lawrence Estuary (SLE) appears to be unique in terms of temperature and oxygen habitat. Over the last few decades, it has been found to be most abundant at 4–5°C, and is now found in waters of 5–6°C (Galbraith et al., 2019). This is associated with a temperature increase of the SLE deep water (>150 m) since 2010 (Galbraith et al., 2019), however, even before 2010, the SLE population was already living under relatively warm conditions when compared to other populations (Gilbert et al., 2005). In terms of environmental oxygen, shrimp from the SLE are exposed to severe hypoxia (17–25% O2 saturation relative to air (% O2 sat. hereafter), Gilbert et al., 2005; Blais et al., 2019), when compared to other populations from deep waters of the wider Gulf of St. Lawrence (GSL) region which are typically exposed to 30–50% O2 sat. (Pillet et al., 2016). In addition, hypoxia in the SLE is expected to worsen as a result of ongoing ocean warming and increased anthropogenic impacts via eutrophication (Dupont-Prinet et al., 2013). Finally, hypoxia is mostly responsible for bottom waters reduction in pH in the SLE (Mucci et al., 2011), and bottom water pH is already as low as 7.75 in the most severely hypoxic regions of shrimp habitat. Thus, bottom waters in the SLE could drop to a pH of 7.40 at the end of the century, because of the superimposition of the ongoing OA and the worsening of hypoxia (Stortini et al., 2017) in coastal waters (RCP 8.5, IPCC, 2014). As such, the shrimp population from the SLE represents an ideal study system to investigate the fate of other stocks of this species in the Atlantic, and more in general the fate of cold-adapted ectotherms species, as they are already exposed to warm, hypoxic, low pH waters.
The aim of our study was to determine the impact of combined OW, OA and hypoxia on the survival and the nutritional and organoleptic qualities on female of the Northern shrimp from the SLE population. Females were specifically selected for this study as they are the main target of the fishery, and because they were shown to be more sensitive when compared to males to hypoxia (Dupont-Prinet et al., 2013). Based on our current understanding of this species biological responses to OW, OA and hypoxia in isolation in adults (Daoud et al., 2007, 2010; Dupont-Prinet et al., 2013; Pillet et al., 2016) and the combined exposure to OW and OA in juveniles and larvae (Daoud et al., 2007; Arnberg et al., 2013, 2018), we hypothesis that the combined exposure to future OW and OA scenarios with hypoxia will affect negatively survival, nutritional and organoleptic qualities of this species in a synergistic manner.
Materials and Methods
Specimen Collection, Transport and Maintenance
Female P. borealis were collected in the SLE near Rimouski (Quebec, Canada; ∼58°36′N, 68°35′W) in May 2018 using a rigid frame trawl (hauls duration of ∼16 min at an average speed of 2.5 knots) at depth 120–150 m from the 22-m CCGS Leim research vessel. Within 4–10 h of capture, shrimp were transported to the Maurice-Lamontagne Institute (MLI), Fisheries and Oceans Canada (Mont-Joli, QC, Canada) in 750 L tanks filled with cold (2 and 3°C), well oxygenated sea water. Once at MLI, shrimp were kept for approx. 8 weeks to adjust to laboratory conditions in 1700 L rectangular tanks, continuously supplied with natural sea water from the SLE pumped from 2 km offshore of the laboratory facilities. Average salinity of the pumped water was 28, which was increased to ∼32 by adding salt (Common salt without additives, K+S Windsor Salt, Pointe-Claire, QC, Canada). Average conditions during this period were 4.5°C, salinity 32, 100% O2 sat. and pH 7.9 (total scale, pHT). Shrimp were fed ad libitum three times a week, with a diet consisting of frozen and finely chopped capelin (Mallotus villosus, Müller, 1776) and shrimp (Pandalus spp.), in equal proportions to mimic their natural diet (Wienberg, 1980). Uneaten food was removed 24 h after each feeding period, while dead individuals and exuviae were removed daily in order to prevent the proliferation of bacteria and ammonia accumulation thus maintaining high quality water levels.
Experimental Design
In order to investigate the effect of combined ocean change drivers on survival, and the nutritional and organoleptic qualities of shrimp, two experimental designs were employed. Experimental design A was used to explore the impact of OW and OA, in isolation and combined, on the traits investigated. Female shrimp were exposed to one of three levels of temperature (2, 6, and 10°C) and two levels of pH (7.75 and 7.4) according to a full orthogonal experimental design composed of six treatments in total. Temperature treatments were selected based on the preferred temperature for this species (2°C, Orr and Sullivan, 2013; Siferd, 2015), the current temperature of the SLE shrimp habitat (6°C) and a +4°C increase scenario predicted to occur by the end of the century (RCP 8.5, IPCC, 2014). Seawater pH levels were chosen to represent current (7.75) (Mucci et al., 2011, 2017) and predicted bottom conditions for 2100 in the SLE (7.40) according to a −0.3/−0.4 pH unit decrease (RCP 8.5 scenario, IPCC, 2014). Additionally, Experimental design B was used to explore the effect of hypoxia superimposed upon current and future combined OW and OA conditions (i.e., the current and future environmental horizons) on the traits investigated. This consisted of two additional treatments, hypoxia (35% O2 sat.) combined with what are considered the most favorable environmental conditions (2°C, pH 7.75) and hypoxia combined with future OW and OA conditions (10°C, pH 7.4). The value of 35% O2 sat. was chosen as a non-lethal (chronic) level of hypoxia commonly encountered by shrimp in the Saint-Lawrence Gulf and Estuary (Dupont-Prinet et al., 2013).
Designs A and B were carried out simultaneously within the same experimental system (see section “Experimental Set Up and Protocol” description below), consisting of a total of eight treatments with two replicate tanks per treatment, designated as follow: low temperature and current pH (2C: 2°C, pH 7.75, 100% O2 sat.), low temperature and low pH (2A: 2°C, pH 7.40, 100% O2 sat.), intermediate temperature and current pH (6C: 6°C, pH 7.75, 100% O2 sat.), intermediate temperature and low pH (6A: 6°C, pH 7.40, 100% O2 sat.), elevated temperature and current pH (10C: 10°C, pH 7.75, 100% O2 sat.), elevated temperature and low pH (10A: 10°C, pH 7.40, 100% O2 sat.), low temperature, current pH and low O2 (2CH: 2°C, pH 7.75, 35% sat.) and elevated temperature, low pH and low O2 (10AH: 10°C, pH 7.40, 35% sat.).
Experimental Set Up and Protocol
Shrimp were held in 16 tanks (duplicate for each treatment) supplied with sea water from two reservoirs (vol. = 750 L) at a constant flow rate of 3.5 L min–1. A thermopump (Gell’Air, Mont-Joli, Canada) maintained each reservoir at a constant temperature of approx. 0 and 10°C, respectively. Each tank was provided with a controller (1/16 DIN Micromega autotune PID Temperature, Omega Engineering inc., Norwalk, CT, United States) and two mixing valves (sv3109, Omega Engineering inc.) to enable mixing of the appropriate proportion of cold and warm water (total 3.5 L min–1) to provide each tank with sea water at the set temperature. Each tank was also equipped with a temperature probe (HRSTD-3-100-A-240-E, Omega Engineering Inc., Norwolk, CT, United States) and a pH probe (1001 modules, IKS Aquastar, Karlsbad, Germany) that allowed for the continuous monitoring and regulation of these environmental parameters through two feedback systems (1/16 DIN Micromega autotune PID Temperature, Omega Engineering inc. and IKS Aquastar, respectively). Additionally, the tanks hosting hypoxic treatments were equipped with an O2 probe (1008 module, IKS Aquastar) that allowed the monitoring and control of % O2 sat. by a feedback system (IKS, Aquastar). The feedback system regulated the addition of pure gaseous CO2 and N2 into each tank’s gas exchange column. A submersible pump (1048, Eheim, Stuttgart, Germany) in each tank allowed water mixing, preventing the formation of physical and chemical gradient. In the tanks hosting hypoxic treatments, the submersible pump was connected to the top of the column in order to minimize the oscillations of % O2 sat by increasing flow rate through the column.
Approx. 70 non-ovigerous females were randomly assigned to each of the 16 large insulated and re-circulated tanks (vol. = 240 L). Starting from the conditions prevailing during the laboratory adjustment period (4.5°C, pH 7.9 and 100% sat.), the experimental tanks were gradually adjusted over 4 days until treatment values were reached. Temperature was increased or decreased by 1.5°C per day, pH was decreased by 0.15 pH units per day, and O2 sat. of 35% was achieved via daily adjustments from 100 to 70%, 50%, 40% and finally 35% at the end of the first, second, third and fourth day, respectively. Once set treatment conditions were reached, shrimp were exposed maintained in these conditions for a total of 30 days. Feeding and cleaning routines were maintained as per the adjustment period detailed above.
At the end of the exposure period, individuals were carefully and rapidly blotted dry with tissue paper and weighted using a precision scale (Mf-300, AandD Company, Tokyo, Japan; ±0.001 g precision). Following weighing, individuals were rapidly placed in individual Ziploc bags, separated by treatment and tank in order to avoid contamination, and frozen at −20°C to be later used for analyses.
Monitoring of Seawater Chemical and Physical Parameters and Characterization of the Carbonate Chemistry
Seawater temperature, % O2 sat., salinity and pHT were measured daily, using, respectively, a thermocouple (HH802U, Omega Ltd., Manchester, United Kingdom), an O2 meter (OXYROB10, Pyrosciences, Aachen, Germany), a conductivity portable meter (Profline Cond3110, WTW, Weilheim, Germany) and a pH meter (914, Metrohm AG, Herisau, Switzerland) calibrated using NBS then TRIS buffers (to convert pH values to the total scale; Dickson et al., 2007), respectively. For the hypoxia treatment, O2 sat. was checked once a week by titration using a method modified from Winkler (1988) described by Jones et al. (1992), and the O2 sat. target for each tank was adjusted to account for the offset between the probes and Winkler titrations.
Seawater samples (vol. = 341 mL) were collected every 4 days in the header tanks and two experimental tanks for total alkalinity (TA) and dissolved inorganic carbon (DIC) determination. Seawater samples were poisoned with 171 μL of saturated HgCl2 solution to stop biological activity from altering the sample and stored in the dark, at stable temperature conditions, pending determination. Total alkalinity was determined by 0.01 N HCl potentiometric titration (Dickson et al., 2007), using an automatic titrator (848 Titrino Plus, Metrohm). DIC determination was conducted by flow injected analysis gas exchange conductivity method, as described by Hall and Aller (1992), using a conductimeter combined to a gas exchange cell (Dionex ICS-1000, Thermo Fisher Scientific, Waltham, MA, United States).
Other carbonate system parameters, including seawater pCO2, bicarbonate ions concentration ([HCO3–]), carbonate ions concentration ([CO32–]) and calcite and aragonite saturation states (Ωcal and Ωara) were calculated using measured seawater pHT, temperature, salinity and TA values using CO2SYS software (Lewis and Wallace, 1998), with dissociation constants from Millero (2010). We then used DIC values to validate our carbonate system calculations (Gattuso and Hansson, 2011). The constants used to calculate Ωcal and Ωara are not applicable at temperatures below 2 °C. Therefore, we discarded samples for which temperature was <2°C for the 2°C treatments. More specifically the number of seawater sampling for the 2C scenario were n = 51, for the 2A scenario were n = 40 and for the 2CH scenario were n = 40 and for the cold temperature, current pH and hypoxia scenario were n = 46. Mean physico-chemical parameters for the duration of the experiment are given in Table 1.

Table 1. Summary of mean values (mean ± SD) physico-chemical parameters of the sea water from different treatments measured and calculated (*): temperature (°C), pH (Total scale), salinity, total alkalinity (TA, mEq kg SW–1), total dissolved carbon dioxide (DIC, mmol kg SW–1), carbon dioxide partial pressure* (pCO2 μatm), bicarbonate concentration* ([HCO], mmol kg SW−1) and carbonate concentration* ([CO], mmol kg SW−1).
Determination of Muscle Mineral Content (Designs A and B)
To determine the content of important minerals (McDowell, 2003) in shrimp muscle, calcium (Ca2+), copper (Cu2+), iron (Fe2+), potassium (K+), magnesium (Mg2+), manganese (Mn2+), strontium (Sr2+) and zinc (Zn2+) concentrations were quantified and expressed as mmol kg–1 dry mass. Muscle samples (n = 5 individuals per tank, 10 per treatment) were prepared as described in Menu-Courey et al. (2019) using plastic tools to avoid any mineral contamination. Samples were thawed and lyophilized using a freeze dryer (Freezone 12 Floor Model, Labconco, Kansas City, MO, United States) for 48 h at −40°C, and then immediately placed in a desiccator in order to keep the samples as dry as possible. Once the samples were dried and ready for analyses, 250 mg of dried muscle was dissected from each sample, weighed with a precision scale (225AC, VWR, Radnor, PA, United States; ±0.00001 g precision), and placed in a 15 mL Falcon tube. Samples were digested with the addition of nitric acid (65–70%, 3 mL) and hydrogen peroxide (25–35%, 1 mL) to each tube which were subsequently vortexed. Falcon tubes were left open 24 h under the extractor hood before they were placed for 2 h in a sonication bath at 45°C (97044-002, VWR) with the caps opened every 15 min in order to enable the degassing of the solution. Digested samples were diluted with nanopure water to reduce the total percentage of total dissolved solids in the samples to within the instrument’s detection limits and to make up sufficient solution for analyses. Mineral concentrations were determined via an inductively coupled plasma analyser (ICP-AES) interfaced to an optical emission spectrometer (Spectro Acros II ICP-OES, SPECTRO Analytical Instrument, Kleve, Germany) at the Geolab (Sudbury, ON, Canada).
Organoleptic Quality Evaluation (Design B Only)
As organoleptic evaluations require a relatively large volume of shrimp, and testing is labor intensive, we limited our comparison to only four treatments, corresponding to those for design B. However, the extensive mortality rate in 10AH made it impossible to have enough live shrimp for organoleptic testing at the end of the exposure period. Therefore, we decided to carry out the test on 10A instead of 10AH as “unfavorable condition,” to insure having a sufficient number of specimens for our tests: n = 21 individuals per tank, 42 per treatment.
Organoleptic evaluations were carried out at the Merinov facility of Gaspé (QC, Canada) where the shrimp were transported frozen inside coolers filled with dry ice in order to ensure that they stayed frozen. In addition, temperature was constantly monitored with a temperature logger (MicroTemp, Madge Tech, Warner, NH, United States) to confirm that shrimp remained completely frozen. At the Merinov laboratory, bags were inspected to detect any damage and stored at −34°C in a freezer (13-986-149, Thermo Fisher Scientific) pending organoleptic assessment. 24 h before organoleptic evaluation, shrimp were thawed at 0–4°C in a refrigerator (FPRU19F8RFC, Frigidaire Professional, Stockholm, Sweden). Shrimp were first generally inspected for general appearance and color criteria according to Quality Index Method (QIM) charter for Northern shrimp (Martinsdóttir et al., 2001). They were then weighed individually using a digital scale (PE360, Mettler-Toledo, Greifensee, Switzerland) and individuals from the same treatment and tank were cooked, based on laboratory preliminary tests carried out to establish the cooking time for each size category: 75 s (4–6 g indiv.), 105 s (7–9 g indiv.) or 120 s (indiv. > 9 g) in 2 L of unsalted boiling water. Shrimp were then scooped, cooled for approx. 1 min in a bowl of icy water, manually peeled and the abdomen tissue (which is the part of the shrimp normally consumed) was sealed in a container and refrigerated until the trays were assembled for assessment. The organoleptic evaluation method followed the guidelines of the XP V09-500 AFNOR Normalization standard on hedonic tests (AFNOR, 2000). Cooked shrimp were maintained at room temperature for 30 min before mounting the trays. Three shrimp were placed in the center of a white dish and panelists received only one set (i.e., one treatment) at a time. The evaluation consisted for each panelist in scoring the color, smell, texture and taste on a nine-level hedonic scale as well as commenting freely their perceptions. All assessments took place in booths that globally respected the NF EN ISO 8589 standard for the design of organoleptic analysis rooms. The light was of daylight type at an average intensity of 780 lux.
Panelists (6), three women and three men, were recruited from Merinov’s staff, and can be defined as an “internal trained panel” as they regularly consumed marine products, had already participated in organoleptic evaluation tests and were specially trained the summer before to evaluate Northern shrimp produced in three different ways: (1) with maturation solution precooking as in the industry, (2) without any precooking treatment, and (3) shrimp from the local market fishmonger. The training allowed panelists to become familiar with the nine-level hedonic form and the vocabulary in organoleptic evaluation for cooked shrimp (Martinsdóttir et al., 2001).
Statistical Analyses
In order to test the effect of GC drivers in isolation and combined on shrimp survival across all treatment tested in designs A and B a one-way ANOVA test was performed, with “treatment” as fixed factor. Differently, in order to test the effect of GC drivers in isolation and combined on shrimp mineral contents for the designs A and B separately, mixed effect models were used (nlme package, Pinheiro et al., 2018). For design A temperature and pH were set as fixed factors, and tank as a random factor to account for any pseudoreplication effect. For design B “horizons” (i.e. current horizon: 2C, future horizon: 10A) and O2 levels (normoxia and hypoxia) were set as fixed factors and tank as a random factor. Tukey HSD tests (Hothorn et al., 2008) were used to conduct post-hoc analyses, when significant effects were evidenced. Normality of distribution and homoscedasticity of variances assumptions were verified using, respectively, the Shapiro’s and Bartlett’s tests, as well as visual analyses of residuals. Survival data were arcsine transformed (Sokal and Rohlf, 1995), and mineral data were log transformed to meet the assumption for normality.
Permutational Manova (Anderson, 2001) were used to test the impact of elevated temperature, low pH and low O2 level (set as treatment: 2C, 2CH, 10C and 10A) on shrimp’s organoleptic attributes: taste, appearance, texture and smell all together. PERMANOVA was performed using Adonis function (Oksanen et al., 2018), with panelist as a blocking factor. A principal component analysis (PCA) was then performed using R to provide a more integrated interpretation of the organoleptic attributes depending on the treatment tested. For that purpose, data were rank transformed and multivariate and univariate normality were tested, respectively, by Mardia Skewness and Mardia Kurtosis tests and Shapiro’s test. Rank data were used on the basis that PCA is a very robust analysis (Tabachnick and Fidell, 2006). Correlation between variables was tested, and no collinearity was detected. The Kaiser-Guttman rule (Jackson, 1993) and the broken stick method (graphic method) were used to determine whether only the first two axes contributed enough to be retained in the analysis. All univariate statistical analyses and assumptions checking were performed using the software R 3.5.1 version (R Core Team, 2018).
As the comments of the panelists were a source of relevant information about the organoleptic quality in addition to the hedonic scale, a word co-occurrence analysis, as a semi-quantitative analysis, was run to seek for any potential pattern in panelist perceptions. As several terms could be used to express the same concept, similar terms were grouped under a new single term. For example, to describe a characteristic color of the shrimp, several terms could be used (such as orange, pink, shrimp color), all terms possessing a positive connotation, in the word analysis, they were all recoded under the name “nice color.” Tropes free software (Molette and Landré, 1994) permitted to determine the three most recurrent keywords among the comments of each attribute categories: taste, smell, texture, and color. This recoding work was done for each of the three most used terms. The occurrence of most recurrent words was then counted by treatment to determine if certain terms occurred more frequently in relation to specific GC drivers in isolation or combination.
Results
Survival
Survival was expressed as survival rate, i.e., the percentage of surviving specimens at the end of the 30 days exposure period, relative to the initial number of specimens per treatment. Results are presented in Figure 1. Survival rates differed significantly among treatments (F1,16 = 38.720, p-value < 0.001). In more detail, survival rates were comparable (approx. 88%) across all other treatments tested, whilst they were significantly lower for the combined elevated temperature and low pH treatment (10A – 67.85%) and for the combined elevated temperature, low pH and low O2 treatment (36.62% – 10AH). Weekly mortality levels were (broadly speaking) relatively evenly distributed across all treatments and weeks of exposure, with the exception of treatments 10A and 10AH where mortalities were higher during the first 2 weeks and then decreased in week 3 and 4, and were in general much higher when compared to the other treatments (Supplementary Table 3).
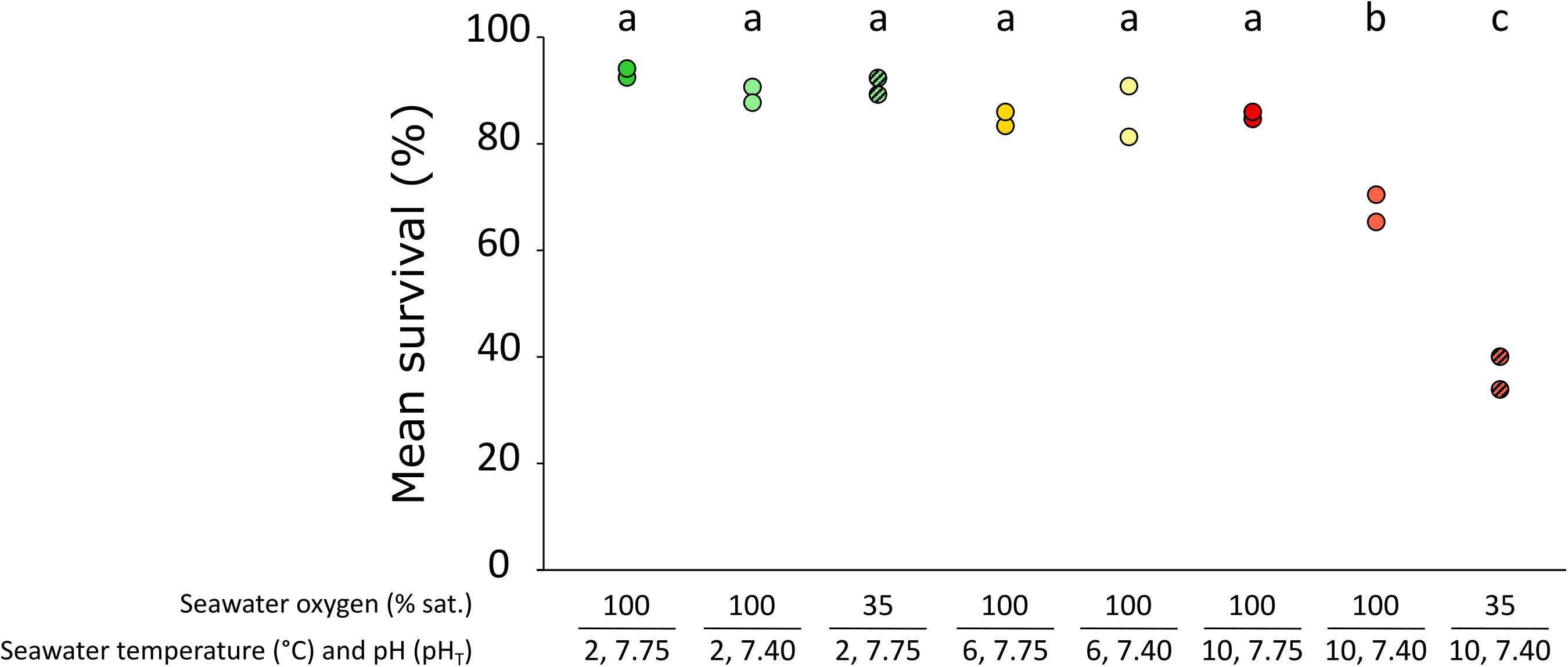
Figure 1. The effect of exposure to elevated temperature, low pH and low oxygen in isolation and combined for 30 days on mean survival (%) of females of the Northern shrimp Pandalus borealis. Treatment symbols correspond to 2C: 2°C, pH 7.75, O2 sat. 100% (dark green); 2A: 2°C, pH 7.4, O2 sat. 100% (light green); 2CH: 2°C, pH 7.75, O2 sat. 35% (light striped green); 6C: 6°C, pH 7.75, O2 sat. 100% (dark orange); 6A: 6°C, pH 7.4, O2 sat. 100% (light orange); 10C: 10°C, pH 7.75, O2 sat. 100% (dark red); 10A: 10°C, pH 7.4, O2 sat. 100% (light red); 10AH: 10°C, pH 7.4, O2 sat. 35% (light striped red). Dots represent mean percentage survival values for each tank. Lower case letters indicate significant differences (p < 0.05) between treatments.
Mineral Content
Concentrations of calcium (Ca2+), copper (Cu2+), iron (Fe2+), magnesium (Mg2+), manganese (Mn2+), strontium (Sr2+), zinc (Zn2+) and potassium (K+) in shrimp muscle (n = 79) are comprehensively reported for all treatments in Supplementary Table 4.
For design A, there was a significant interaction between temperature and pH for [Cu2+] (F2,6 = 6.547, p-value = 0.031; Figure 2). [Cu2+] was significantly lower at low pH only at 6°C, while there was no effect of low pH at 2 and 10°C, and temperature had no effect at control pH. There was no interaction for other cations, but significant effects of elevated temperature were detected, whilst there was no effect of pH. In fact, most of the cation’s concentrations were highest at 10°C. This included [K+], [Mg2+] and [Zn2+], which were significantly higher at 10°C when compared at 2 and 6°C (minimum F1,6 = 6.793, p-value = 0.029), increasing by 12, 12, and 21%, respectively, at 10°C when compared to 2°C. [Sr2+] significantly increased by 25% at 10°C when compared to 6 and 2°C (F1,6 = 5.277, p-value = 0.048, post hoc test p > 0.05).
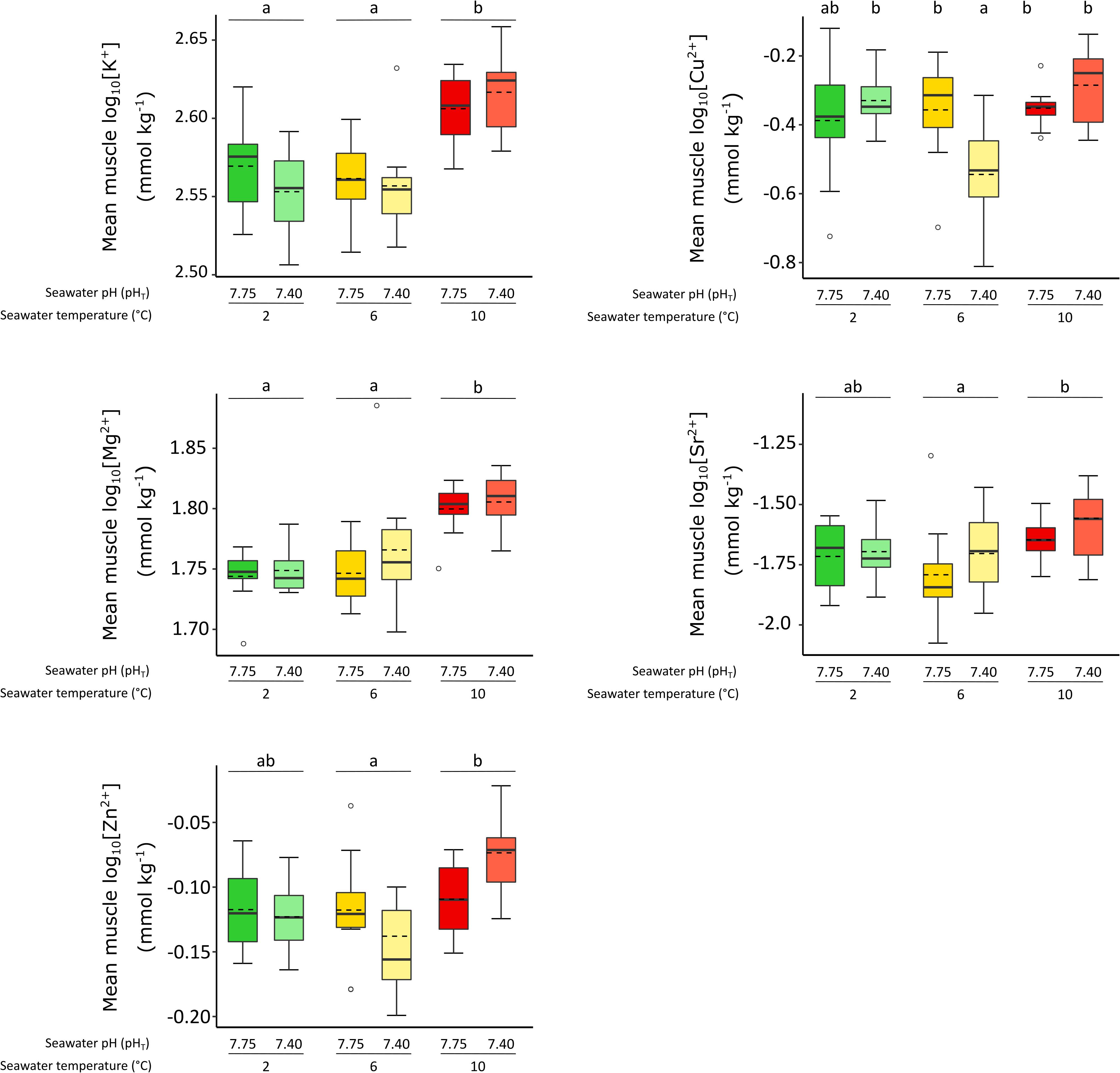
Figure 2. The effect of exposure to elevated temperature and low pH in isolation and combined for 30 days on mean muscle log10 [K+], [Cu2+], [Mg2+], [Sr2+], [Zn2+] (mmol kg–1 ± SE) of female P. borealis. Box plots represent different treatment, with plain lines indicating the median, dotted lines representing the mean. Lower case letters identify significant differences (p < 0.05) between treatments, empty circles represent extreme values exceeding the 95% quantile for each treatment.
For design B, higher [K+], [Fe2+] and [Mg2+] were found in shrimp exposed to the future horizon scenarios (combined elevated temperature and low pH) both under normoxic and hypoxic conditions (minimum F1,4 = 9.810, p-value = 0.035, respectively), when compared to current conditions (Figure 3). [K+], [Fe2+] and [Mg2+], respectively, increased by 12, 30, and 9% under future conditions compare to the 2°C scenarios. There was no effect of hypoxia on the content of any of the minerals investigated.
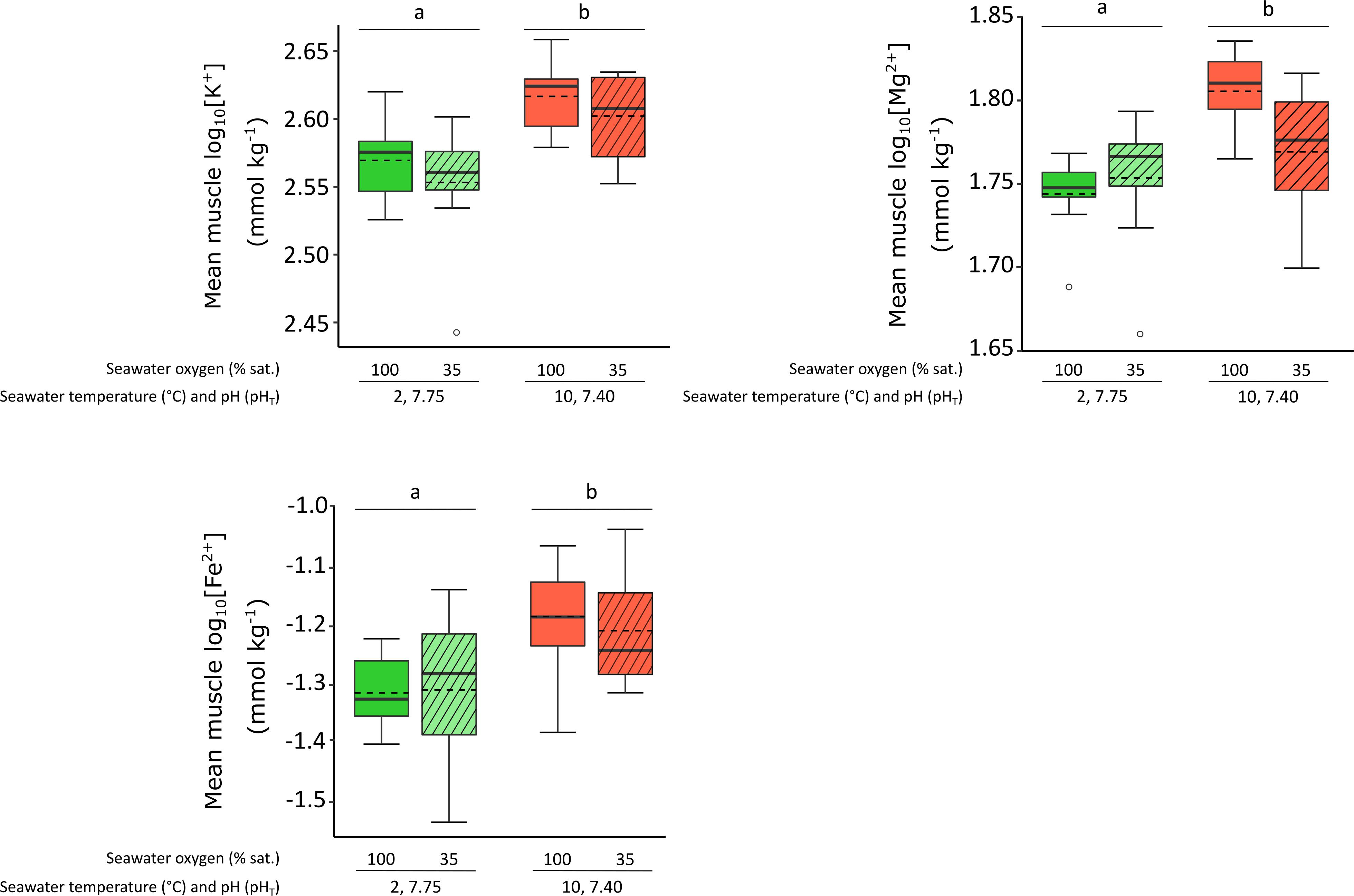
Figure 3. The effect of exposure to elevated temperature and low pH combined (i.e., current and future environmental horizon scenarios), in isolation and combined with low oxygen on mean muscle log10 [K+], [Mg2 +] and [Fe2 +] (mmol kg−1± SE) of female P. borealis. Box plots represent treatment, with plain lines representing the median, dotted lines representing the mean. Lower case letters identify significant differences (p < 0.05) between treatments, empty circles represent extreme values exceeding the 95% quantile for each treatment.
Organoleptic Quality
Rankings for each organoleptic attribute (smell, texture, taste, and appearance) are presented in Table 2. Trained panelists revealed that organoleptic attributes were not significantly affected by elevated temperature, low pH or low O2 (maximum F168,1 = 0.785, p = 0.306), with no significant differences found between replicates (F168,1 = 0.308, p = 0.713) within each tested treatment.
Figure 4 shows the absence of any pattern in organoleptic attributes with no specific spatial grouping of all samples according to the exposure treatment. The two selected principal components (PC) explained 81.86% of the total variance. The first PC explained 52.38% and the second PC explained 29.48% of the variance.
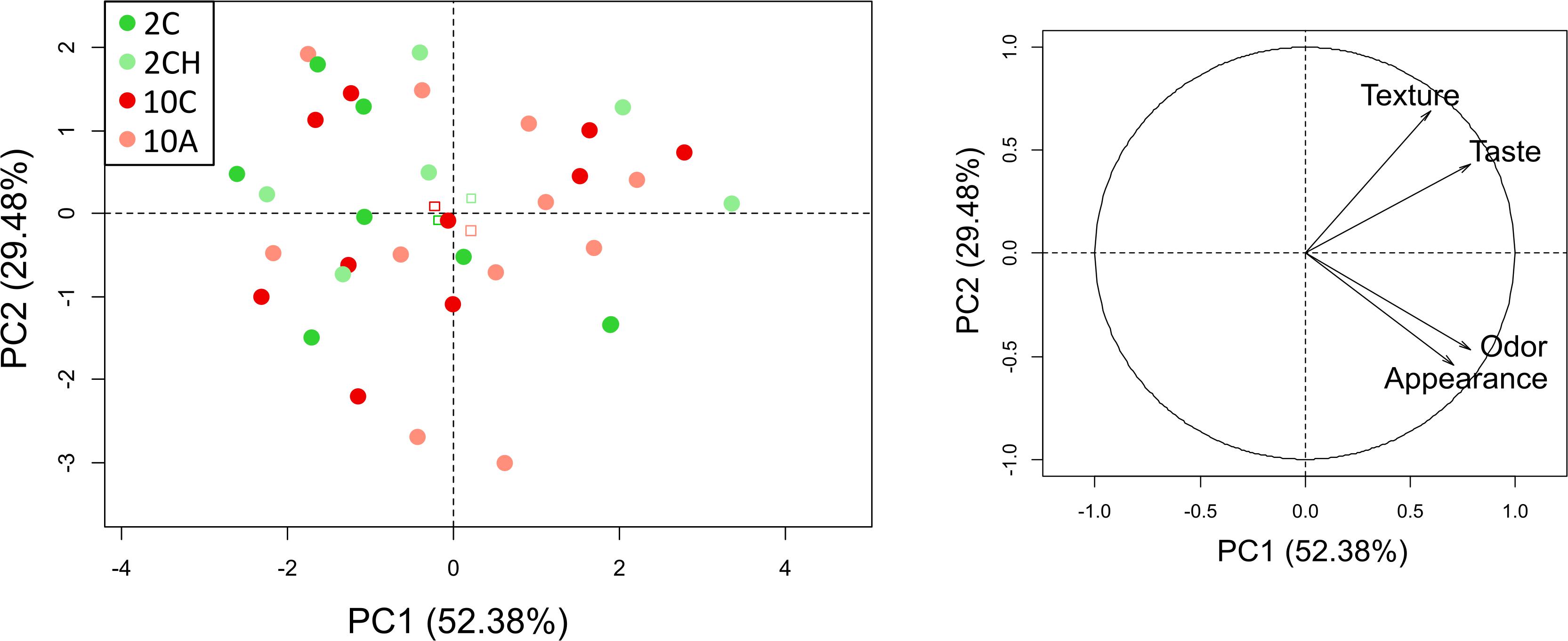
Figure 4. Distribution of the samples organoleptic attributes on the basis of the first two principal components according to their treatment. Treatment symbols correspond to 2C: 2°C, pH 7.75, O2 sat. 100% (dark green); 2CH: 2°C, pH 7.75, O2 sat. 35% (light green); 10C: 10°C, pH 7.75, O2 sat. 100% (dark red); 10A: 10°C, pH 7.4, O2 sat. 100% (light red). Squares represent barycenters for each treatment. Then correlation circle on the right show the distribution of the explanatory variables in the space defined by the 2 axes.
Word occurrence analysis presented in Table 2 showed the absence of any pattern. The characteristic “sweetness” of the product was pointed out for all treatments, whilst the absence of the terms “watery”, “phosphated” and “salted” (typically caused by the precooking of shrimp in a maturation solution) was only noted for the current pH and cold temperature scenario. The color, whether characteristic or uneven, was reported equally for all the treatments. The marine fresh smell of shrimp was reported for all treatments equally, while ammonia smell was reported twice for the cold temperature and current pH and the elevated temperature and low pH scenarios. The texture was described more times as “pasty” in individuals exposed to 10°C treatments, while the samples firmness was reported evenly in shrimp across all treatments.
Discussion
In this study we show that exposure to OW and OA combined, and even more so when these two global drivers co-occur with hypoxia, strongly reduces the survival of the Northern shrimp P. borealis, whilst it does not impact significantly its organoleptic properties. Shrimp muscle mineral content increases overall under elevated temperatures but is not affected by OA or hypoxia. Altogether, our results represent good news, as the Northern shrimp will likely remain a tasty and healthy food source under predicted future environmental scenarios. However, the tremendous decrease in survival we report here represents an important cause of worry for the conservation of this species, and for its fisheries. As such, our results will be discussed within the context of the nutritional and economic importance of the Northern shrimp in the future ocean.
Survival Decreases Severely Under Elevated Temperature, Low pH and Hypoxia
Female shrimp survival decreases approx. by a factor of 2.5 when exposed to combined elevated temperature, low pH and hypoxia, compared to scenarios involving the cold and intermediate temperatures tested here, as well as the elevated temperature and current pH scenario. Furthermore, exposure to elevated temperature and low pH, under normoxic conditions, resulted in decreased survival rates (1.3 times lower) when compared to low and intermediate temperatures scenarios. Negative effects of elevated temperature and decreased pH (in isolation and combined) on survival rates have already been reported for the Northern shrimp (Dupont et al., 2014; Arnberg et al., 2018). Dupont et al. (2014) showed that a 3-week exposure to seawater pH 7.5/pCO2 1368 μatm decreased adult survival by a factor of 1.6 compared to pH 8.0/pCO2 759 μatm conditions in the Gullmarsfjord (Sweden) population at 11°C: it is important to note that in the Gullmarsfjord the mean summer temperature (June to August) is approx. 13 ± 7°C (Dorey et al., 2013). Similarly, Arnberg et al. (2018) showed that a 27-day exposure to combined OA and OW (6.5°C, pH 8.1 versus 9.5°C, pH 7.6) decreased larvae survival by a factor of 1.3 in the Lysefjorden (Norway) population. Altogether, our results are comparable to those reported by Dupont et al. (2014) and Arnberg et al. (2018). In addition, we show that hypoxia represents a major threat to shrimp survival in the future ocean, i.e., when co-occurring with future OA and OW. Hypoxia is an increasing phenomenon, occurring now in more localities, more frequently, and for longer periods of time (Diaz, 2001). Reduced survival in shrimp exposed to the combined elevated temperature, low pH and hypoxia confirms synergistic interaction between these drivers (Côté et al., 2016).
Although OW alone (10°C) did not significantly negatively affected shrimp survival compared to 2 and 6°C, shrimp appear nonetheless to be very sensitive to OA acidification and hypoxia at this temperature. This ultimately suggests that the threshold of thermal sensitivity of this population is likely closer to 10°C than to 6°C. This idea is further supported by considering that the optimal temperature range for the species in the field is −1.6 to 8°C (Garcia, 2007).
It is important also to notice that survival was slightly lower for treatments at 6°C when compared to those at 2°C; however, no significant difference was found between these two temperatures. Whilst this could result from the low power of our experiment (for mortality data only), as we used two tanks per treatment, it also should be noted that other studies have also revealed a good tolerance of shrimp to 6°C (Chabot unpublished data).
Further to our discussion above, it is important to consider that the Swedish population examined by Dupont et al. (2014) is subjected only to seasonal hypoxia and annual warmer temperature but similar pH conditions (Dorey et al., 2013), when compared to the SLE population which faces chronic hypoxia in bottom water (Dupont-Prinet et al., 2013). Thus comparing these two different, geographically distant and genetically separated, populations (Jorde et al., 2015) suggests the presence of local adaptation or of signs of long-term acclimatization to their native habitat conditions.
Finally, our results confirm the importance of reporting survival information in experiments attempting to address the fate of species of commercial interest. This will (in time) help defining the likely trajectory of the future distribution and biomass of different populations of P. borealis, and so on the potential implications for its conservation and fisheries (Cooley and Doney, 2009; Fernandes et al., 2017).
Shrimps’ Mineral Content Increase With Increasing Temperature
Minerals have several biological functions in animals, as they are involved in the structure (e.g., maintenance and formation of bones, teeth and soft tissues), physiological functions (e.g., blood clotting, component of respiratory pigments) and cell reaction and regulation (e.g., enzymes cofactors, components of hormones and vitamins) (McDowell, 2003). Minerals are required for human dietary intake because they participate in many biological processes that support and maintain a healthy life (Hirayama et al., 2010; Yatoo et al., 2013). Among these essential minerals, some are considered as macroelements (incl. Ca2+, Mg2+ and K+), whilst others are defined as microelements (incl. Zn2+, Cu2+, Fe2+ and Mn2+). The most abundant minerals in P. borealis muscle are, respectively, K+, Mg2+ and Ca2+. Mean [Ca2+], [Fe2+], [Mn2+] and [Zn2+] values reported here agree with values found in the broader literature for mineral contents in other decapod shrimp species (e.g., Balkas et al., 1982; Pourang and Amini, 2001; Adeyeye and Adubiaro, 2004; Cerezo Valverde et al., 2015). However, mean values for [Cu2+] and [Mg2+] appear to be 2.5 times higher in our study, compared to concentrations reported for other shrimp species (Adeyeye and Adubiaro, 2004; Oksuz et al., 2009, respectively), and for [K+] 4 times higher compared to the concentration found in muscle of the deep water rose shrimp Parapenaeus longirostris (Lucas, 1846; Oksuz et al., 2009).
Our work showed that shrimp muscle mineral content is globally greater at the highest temperature we tested (i.e., 10°C), whilst exposure to low pH and low O2 does not affect significantly mineral content. With increasing temperature metabolic demand increases (Angilletta, 2009), likely increasing protein synthesis (Carter and Houlihan, 2001) and disturbing osmoregulatory capacities (Vlasblom et al., 1977). This pathway could partly explain why mineral contents were greater at 10°C in our study. Severe hypoxia (<35% O2 sat.) was shown to reduce aerobic scope: i.e., the difference between maximum metabolic rates and standard metabolic rates (Claireaux and Chabot, 2016), including in P. borealis (Dupont-Prinet et al., 2013). This could explain why at 10°C under hypoxic conditions close to those of Dupont-Prinet et al. (2013), we do not see a significant increase in mineral contents, via the presence of an antagonistic relationship between elevated temperature and hypoxia. Finally, as under combined elevated temperature, low pH and low O2 conditions shrimp can experience simultaneously internal acidosis, hypoxemia and a metabolic depression (Burnett, 1997; Pörtner and Lanning, 2009), ion incorporation activity could have been depressed.
From a nutritional perspective, as the mineral content increases with temperature, a smaller portion of shrimp than the 25–30 g recommended quantity will provide a sufficient amount of minerals, respecting the daily recommendations intake for K+, Cu2+, Fe2+, Sr2+ and Zn2+ (Raymont et al., 1967; World Health Organization [WHO], 1996). In more details, under future environmental conditions a 30 g portion of shelled shrimp will contribute, respectively, to 36.5, 16.1, 13.7, 12.7, 11.1, 3.9, 0.78, and 0.42% of daily [Cu2+], [Mg2+], [Sr2+], [K+], [Zn2+], [Ca2+], [Fe2+], and [Mn2+] requirement. Within the context of future GC, our results show that the Northern shrimp will maintain a good nutritional value for human consumption, continuing to represent an excellent source of minerals.
Shrimp Organoleptic Quality Remained Unchanged Under Combined Global Change Ocean Drivers
Pandalus borealis organoleptic attributes are not affected by GC drivers (in isolation or combined) at levels predicted to occur by the year 2100 (IPCC, 2014), according to a panel of trained tasters. Furthermore, word occurrence analysis of panelists’ comments reveals that the description of shrimp exposed to the combined OW, OA and hypoxia scenario were as good as those for the other scenarios tested, including for the most favorable environmental conditions. Our results differ from those of Dupont et al. (2014), who showed a negative effect of low pH on organoleptic quality (e.g., taste and appearance) of P. borealis. The discrepancy between these two studies may be related to a number of factors. Differences in the experimental design and protocol, and actual tested variables may have played an important role in the different effects reported. First, in our study, shrimp were frozen and sent for organoleptic assessment, while in Dupont et al. (2014) shrimp were immediately prepared after the exposure period. Furthermore, preparation of the shrimp differed between the two studies, cooking time was 3 min in salted water in Dupont et al. (2014), whilst shrimp were cooked between 1′15 and 2′00 min (according to body mass) in non salted water in our study. Sarower et al. (2012) showed that cooking time, and the use of salt, can increase nitrogen compounds, contributing to the intensification of differences in organoleptic attributes. Finally, diet provided to the shrimp during the exposure time differed between the two experiments. In Dupont et al. (2014), shrimp were fed ad libitum on chopped herring Clupea harengus, blue mussel Mytilus edulis, artemia Artemia nauplii, and Marine Flake (New Era, Thorne, United Kingdom) for an exposure period of 21 days, whilst in our experiment they were fed ad libitum on frozen and finely chopped capelin and shrimp: our diet mimicking shrimp’s natural diet (Wienberg, 1980) for 30 days of exposure. As taste is strongly influenced by the food consumed by an animal, the different diets provided could further help explaining the different results obtained in our study.
Finally, it is important to consider that as a result of local adaptation and/or long-term acclimatization, the biochemical properties and plasticity to GC drivers (Lardies et al., 2010; Calosi et al., 2017), and thus the organoleptic properties, of shrimp from the populations investigated by Dupont et al. (2014) and that in our study may greatly differ. Genetic diversification and resulting difference in physiological adaptation between these populations could further contribute to explain the different biochemical baselines and responses to environmental factors reported.
Conclusion
Organoleptic quality of P. borealis will likely remain unchanged according to trained panelists under future predicted GC scenarios of OW, OA and hypoxia. From a nutritional perspective, shrimp mineral contents are greater at the highest temperature tested (i.e., 10°C), whilst exposure to low pH and low O2 level do not affect these parameters. Consequently, the future nutritional value and organoleptic quality should largely remain unchanged within the context of combined future ocean change drivers, and thus shrimp still constitute a great source of minerals for human consumption. However, these encouraging results for seafood quality are outweighed by the tremendous reduction in survival observed for females under predicted future GC. The level of reduction in survival may cause negative repercussions for population recruitment and lead to biomass reduction, particularly in the SLE where warming will be accompanied by worsening of O2 and pH levels. Assuming our survival rates realistic in the wild, future environmental changes may cause reduction in shrimp stocks exposed to environmental temperature approaching 10°C, as already observed in the Gulf of Maine (Hunter et al., 2007). Ultimately, P. borealis distribution and abundance patterns in the North West Atlantic may change as a result of populations distributional shifts to follow favorable temperature, as reported in Greenland (Garcia, 2007) and the Western Barents Sea (ICES, 2019). In addition, local extinctions could particularly interest populations in the southern range of this species: see at this regard the introduction of a fishing moratorium in Maine due to the extreme reduction in shrimp abundance (Hunter et al., 2007) and reduction in shrimp in the Fundy Bay area (observations reported by local fishermen R. King and K. Leonard). Finally, higher mortalities and distribution shifts could induce local biomass reductions, leading to economic and social implications for fishery-based coastal communities, as predicted for example for the fin-fish and shellfish fishery under GC scenarios for the United Kingdom (Fernandes et al., 2017).
Data Availability Statement
All data from this article will be available on Pangaea database, under OA-ICC data compilation, with a digital object identifier DOI assigned later.
Author Contributions
FN, DC, and PC conceived the general experimental design, and supported MC in finalizing the design and protocol used. DC provided the installations for the experimental setups and the live shrimp for the study. MC set up the experimental system with help from DC. MC and EG carried out the experiment. PC provided the installations for the laboratory and alkalinity analyses and MC carried them out. LL organized and train the panel and MC, as well as supervised the organoleptic test. MC completed the statistical analyses with support from FN, DC, LL, and PC. MC wrote the first draft of the manuscript, and all authors contributed to the final version of the manuscript.
Funding
This work was supported by an OURANOS grant (554023) to PC, FN, and DC, a FIR UQAR grant and a Canada Foundation for Innovation grant to PC, and DFO Strategic Program for Ecosystem-Based Research and Advice grant, as well as Aquatic Climate Change Adaptation Services Program grant, both awarded to DC. MC and EG were supported by a MITACS-Merinov Accelerate grant and a MITACS-Ouranos Accelerate grant (IT010005), respectively, and by a Natural Sciences and Engineering Research Council of Canada (NSERC) Discovery grant (RGPIN-2015-06500 and RGPIN-2020-05627) all awarded to PC.
Conflict of Interest
The authors declare that the research was conducted in the absence of any commercial or financial relationships that could be construed as a potential conflict of interest.
Acknowledgments
We wish to thank C. Anderson, C. Aubé, L. Beaudin, N. Bertrand-Maltais, T. Hansen, J.-P. Lapierre, and D. Picard for technical support during the experiment at the Maurice Lamontagne Institute (DFO, Canada). We are grateful to Merinov laboratory staff A. Provençal, M.-É. Carbonneau and D. Ouellet for their help in organoleptic assessment and vitamin extractions. We finally want to thank S. Dupont for information and discussion about the Northern shrimp population of Gullmarsfjord. Finally, we are grateful to D. Small for the thorough linguistic revision of our manuscript. MC, EG, FN, and PC are active members of the inter-institutional strategic research network Québec-Océan; MC, EG, and PC are active members of the inter-institutional strategic research network Quebec Center for Biodiversity Science, and FN and DC are member of the Ressources Aquatiques Québec inter-institutional strategic research network.
Supplementary Material
The Supplementary Material for this article can be found online at: https://www.frontiersin.org/articles/10.3389/fmars.2020.00611/full#supplementary-material
References
Ab Lah, R., Smith, J., Savins, D., Dowell, A., Bucher, D., and Benkendorff, K. (2017). Investigation of nutritional properties of three species of marine turban snails for human consumption. Food Sci. Nutr. 5, 14–30. doi: 10.1002/fsn3.360
Adeyeye, E. I., and Adubiaro, H. O. (2004). Chemical composition of shell and flesh of three prawn samples from Lagos lagoon. J. Sci. Food Agric. 84, 411–414. doi: 10.1002/jsfa.1649
AFNOR (2000). Analyse Sensorielle, Méthodologie, Directives Générales Pour la Réalisation D’épreuves Hédoniques en Laboratoire D’évaluation Sensorielle Ou En Salle En Conditions Contrôlées Impliquant Des Consommateurs. XP V 09-500. Paris: Association Française de Normalisation.
Anacleto, P., Maulvault, A. L., Bandarra, N. M., Repolho, T., Nunes, M. L., Rosa, R., et al. (2014). Effect of warming on protein, glycogen and fatty acid content of native and invasive clams. Food Res. Int. 64, 439–445. doi: 10.1016/j.foodres.2014.07.023
Anderson, M. J. (2001). Permutation tests for univariate or multivariate analysis of variance and regression. Can. J. Fish. Aquat. Sci. 58, 626–639. doi: 10.1139/f01-004
Angilletta, M. J. Jr. (2009). Thermal Adaptation: A Theoretical and Empirical Synthesis. Oxford: Oxford University Press.
Arnberg, M., Calosi, P., Spicer, J. I., Taban, I. C., Bamber, S. D., Westerlund, S., et al. (2018). Effects of oil and global environmental drivers on two keystone marine invertebrates. Sci. Rep. 8:17380.
Arnberg, M., Calosi, P., Spicer, J. I., Tandberg, A. H. S., Nilsen, M., Westerlund, S., et al. (2013). Elevated temperature elicits greater effects than decreased pH on the development, feeding and metabolism of northern shrimp (Pandalus borealis) larvae. Mar. Biol. 160, 2037–2048. doi: 10.1007/s00227-012-2072-9
Balkas, T. I., Tug̈rul, S., and Salihoğlu, I. (1982). Trace metal levels in fish and crustacea from Northeastern Mediterranean coastal waters. Mar. Environ. Res. 6, 281–289. doi: 10.1016/0141-1136(82)90042-3
Blais, M., Galbraith, P. S., Plourde, S., Scarratt, M., Devine, L., and Lehoux, C. (2019). Chemical and biological oceanographic conditions in the estuary and gulf of St. Lawrence during 2017. DFO Can. Sci. Advis. Sec. Res. 9, 4–56.
Bourdages, H., and Marquis, M. (2019). Assessment of Northern Shrimp Stocks in the Estuary and Gulf of St. Lawrence in 2017: Commercial Fishery Data. Khalini: DFO.
Burnett, L. E. (1997). The challenges of living in hypoxic and hypercapnic aquatic environments. Am. Zool. 37, 633–640. doi: 10.1093/icb/37.6.633
Caldeira, K., and Wickett, M. E. (2003). Oceanography: anthropogenic carbon and ocean pH. Nature 425:365. doi: 10.1038/425365a
Calosi, P., Melatunan, S., Turner, L. M., Artioli, Y., Davidson, R. L., Byrne, J. J., et al. (2017). Regional adaptation defines sensitivity to future ocean acidification. Nat. Commun. 8:13994.
Cerezo Valverde, J., Tomás Vidal, A., Martínez-Llorens, S., Pascual, M., Gairín, J., Estefanell, J., et al. (2015). Selection of marine species and meals for cephalopod feeding based on their essential mineral composition. Aquac. Nutr. 21, 726–739. doi: 10.1111/anu.12195
Claireaux, G., and Chabot, D. (2016). Responses by fishes to environmental hypoxia: integration through Fry’s concept of aerobic metabolic scope. J. Fish Biol. 88, 232–251. doi: 10.1111/jfb.12833
Cooley, S. R., and Doney, S. C. (2009). Anticipating ocean acidification’s economic consequences for commercial fisheries. Environ. Res. Lett. 4:024007. doi: 10.1088/1748-9326/4/2/024007
Côté, I. M., Darling, E. S., and Brown, C. J. (2016). Interactions among ecosystem stressors and their importance in conservation. Proc. R. Soc. B Biol. Sci. 283:20152592. doi: 10.1098/rspb.2015.2592
Daoud, D., Chabot, D., Audet, C., and Lambert, Y. (2007). Temperature induced variation in oxygen consumption of juvenile and adult stages of the northern shrimp, Pandalus borealis. J. Exp. Mar. Biol. Ecol. 347, 30–40. doi: 10.1016/j.jembe.2007.02.013
Daoud, D., Lambert, Y., Audet, C., and Chabot, D. (2010). Size and temperature-dependent variations in intermolt duration and size increment at molt of Northern Shrimp, Pandalus borealis. Mar. Biol. 157, 2655–2666. doi: 10.1007/s00227-010-1526-1
DFO (2016). An Assessment of Northern Shrimp (Pandalus borealis) in Shrimp Fishing Areas 4-6 and of Striped Shrimp (Pandalus montagui) in Shrimp Fishing Area 4 in 2015. Canadian Science Advisory Secretariat Science Advisory Report 2016/028. Khalini: DFO.
DFO (2018a). 2017 Value of Atlantic Coast Commercial Landings, by Region (thousand dollars). Available online at: http://www.dfo-mpo.gc.ca/stats/commercial/land-debarq/sea-maritimes/s2017av-eng.htm (accessed July 12, 2020).
DFO (2018b). Assessment of Northern Shrimp stocks in the Estuary and Gulf of St. Lawrence in 2017. DFO Can. Sci. Advis. Sec. Sci. Advis. Rep., 2018/015. Khalini: DFO.
Diaz, R. J. (2001). Overview of hypoxia around the world. J. Environ. Qual. 30, 275–281. doi: 10.2134/jeq2001.302275x
Dickson, A. G., Sabine, C. L., and Christian, J. L. (2007). Guide to Best Practices for Ocean CO2 Measurments 2007. Canada: North Pacific Marine Science Organization.
Dorey, N., Lançon, P., Thorndyke, M., and Dupont, S. (2013). Assessing physiological tipping point of sea urchin larvae exposed to a broad range of pH. Glob. Change Biol. 19, 3355–3367.
Dupont, S., Hall, E., Calosi, P., and Lundve, B. (2014). First evidence of altered sensory quality in a shellfish exposed to decreased pH relevant to ocean acidification. J. Shellfish Res. 33, 857–861. doi: 10.2983/035.033.0320
Dupont-Prinet, A., Pillet, M., Chabot, D., Hansen, T., Tremblay, R., and Audet, C. (2013). Northern shrimp (Pandalus borealis) oxygen consumption and metabolic enzyme activities are severely constrained by hypoxia in the Estuary and Gulf of St. Lawrence. J. Exp. Mar. Biol. Ecol. 448, 298–307. doi: 10.1016/j.jembe.2013.07.019
Dworjanyn, S. A., and Byrne, M. (2018). Impacts of ocean acidification on sea urchin growth across the juvenile to mature adult life-stage transition is mitigated by warming. Proc. R. Soc. B Biol. Sci. 285:20172684. doi: 10.1098/rspb.2017.2684
FAO (2018). La Situation Mondiale Des Pêches et De L’aquaculture 2018. Atteindre les Objectifs de Développement Durable. Rome: FAO.
Fernandes, J. A., Papathanasopoulou, E., Hattam, C., Queirós, A. M., Cheung, W. W., Yool, A., et al. (2017). Estimating the ecological, economic and social impacts of ocean acidification and warming on UK fisheries. Fish Fish. 18, 389–411. doi: 10.1111/faf.12183
Galbraith, P., Chassé, J., Caverhill, C., Nicot, P., Gilbert, D., Pettigrew, B., et al. (2019). Physical Oceanographic Conditions in the Gulf of St. Lawrence in 2018. DFO Can. Sci. Advis. Sec. Res. Doc., (2017/044). Khalini: DFO, 5–91.
Garcia, E. G. (2007). The northern shrimp (Pandalus borealis) offshore fishery in the Northeast Atlantic. Adv. Mar. Biol. 52, 147–266. doi: 10.1016/s0065-2881(06)52002-4
Gilbert, D., Sundby, B., Gobeil, C., Mucci, A., and Tremblay, G. H. (2005). A seventy-two-year record of diminishing deep-water oxygen in the St. Lawrence estuary: the northwest Atlantic connection. Limnol. Oceanogr. 50, 1654–1666. doi: 10.4319/lo.2005.50.5.1654
Gruber, N. (2011). Warming up, turning sour, losing breath: ocean biogeochemistry under global change. Philos. Trans. R. Soc. A Math. Phys. Eng. Sci. 369, 1980–1996. doi: 10.1098/rsta.2011.0003
Hall, P. O. J., and Aller, R. C. (1992). Rapid, small-volume, flow injection analysis for ΣCO2 and NH4+ in marine and freshwaters. Limnology 37, 1113–1119. doi: 10.4319/lo.1992.37.5.1113
Hardie, D., Covey, M., Nickerson, K., and King, M. (2015). Scotian Shelf Shrimp 2014-2015. Khalini: DFO, 5–44.
Hilmi, N., Allemand, D., Dupont, S., Safa, A., Haraldsson, G., Nunes, P. A., et al. (2013). Towards improved socio-economic assessments of ocean acidification’s impacts. Mar. Biol. 160, 1773–1787. doi: 10.1007/s00227-012-2031-5
Hirayama, F., Lee, A. H., Oura, A., Mori, M., Hiramatsu, N., and Taniguchi, H. (2010). Dietary intake of six minerals in relation to the risk of chronic obstructive pulmonary disease. Asia Pac. J. Clin. Nutr. 19, 572–577.
Hothorn, T., Bretz, F., and Westfall, P. (2008). Simultaneous inference in general parametric models. Biometr. J. J. Math. Methods Biosci. 50, 346–363. doi: 10.1002/bimj.200810425
Hunter, M., Glenn, R., Whitmore, K., McBane, C., Idoine, J., and Spear, B. (2007). ASSESSMENT REPORT. Arlington: Atlantic States Marine Fisheries Commission.
ICES (2019). Pandalus Assessment Group Meeting, 08 to 13 November 2019 Havforskningsinstituttet (IMR). NAFO SCS Doc. 19/24 Serial No. N7026. Tromsø: ICES.
IPCC (2014). “Climate change 2014 synthesis report - IPCC,” in Proceedingsof the Climate Change 2014: Synthesis Report. Contribution of Working Groups I, II and III to the Fifth Assessment Report of the Intergovernmental Panel on Climate Change (Geneva: IPCC), 2–26.
Jackson, D. A. (1993). Stopping rules in principal components analysis: a comparison of heuristical and statistical approaches. Ecology 74, 2204–2214. doi: 10.2307/1939574
Jones, E. P., Zemlyak, F., and Stewart, P. (1992). Operating Manual for the Bedford Institute of Oceanography Automated Dissolved Oxygen Titration System. Dartmouth: Bedford Institute of Oceanography, 6–51.
Jorde, P. E., Søvik, G., Westgaard, J. I., Albretsen, J., André, C., Hvingel, C., et al. (2015). Genetically distinct populations of northern shrimp, Pandalus borealis, in the North Atlantic: adaptation to different temperatures as an isolation factor. Mol. Ecol. 24, 1742–1757. doi: 10.1111/mec.13158
Lardies, M. A., Arias, M. B., and Bacigalupe, L. D. (2010). Phenotypic covariance matrix in life-history traits along a latitudinal gradient: a study case in a geographically widespread crab on the coast of Chile. Mar. Ecol. Prog. Ser. 412, 179–187. doi: 10.3354/meps08694
Lemasson, A. J., Kuri, V., Hall-Spencer, J. M., Fletcher, S., Moate, R., and Knights, A. M. (2017). Sensory qualities of oysters unaltered by a short exposure to combined elevated pCO2 and temperature. Front. Mar. Sci. 4:352. doi: 10.3389/fmars.2017.00352
Lewis, E., and Wallace, D. W. R. (1998). Program Developed for CO2 System Calculations. Oak Ridge, TN: Oak Ridge National Laboratory, U.S. Department.
López, I. R., Kalman, J., Vale, C., and Blasco, J. (2010). Influence of sediment acidification on the bioaccumulation of metals in Ruditapes philippinarum. Environ. Sci. Pollut. Res. 17, 1519–1528. doi: 10.1007/s11356-010-0338-7
Martinsdóttir, E., Sveinsdóttir, K., Luten, J., Schelvis-Smit, R., and Hyldig, G. (2001). Reference Manual for the Fish Sector : Sensory Evaluation of Fish Freshness. Ijmuiden: QIM Eurofish.
Menu-Courey, K., Noisette, F., Piedalue, S., Daoud, D., Blair, T., Blier, P. U., et al. (2019). Energy metabolism and survival of the juvenile recruits of the American lobster (Homarus americanus) exposed to a gradient of elevated seawater pCO2. Mar. Environ. Res. 143, 111–123. doi: 10.1016/j.marenvres.2018.10.002
Millero, F. J. (2010). History of the equation of state of seawater. Oceanography 23, 18–33. doi: 10.5670/oceanog.2010.21
Molette, P., and Landré, A. (1994). Logiciel TROPES. Available online at: http://www.tropes.fr (accessed February 12, 2020).
Mucci, A., Levasseur, M., Gratton, Y., Martias, C., Scarratt, M., Gilbert, D., et al. (2017). Tidally induced variations of pH at the head of the Laurentian Channel. Can. J. Fish. Aquat. Sci. 75, 1128–1141. doi: 10.1139/cjfas-2017-0007
Mucci, A., Starr, M., Gilbert, D., and Sundby, B. (2011). Acidification of lower St. Lawrence Estuary bottom waters. Atmos. Ocean 49, 206–218. doi: 10.1080/07055900.2011.599265
Oksanen, J., Blanchet, F. G., Friendly, M., Kindt, R., Legendre, P., McGlinn, D., et al. (2018). vegan: Community Ecology Package. R package version 2.5-3.
Oksuz, A., Ozyilmaz, A., Aktas, M., Gercek, G., and Motte, J. (2009). A comparative study on proximate, mineral and fatty acid compositions of deep seawater rose shrimp (Parapenaeus longirostris, Lucas 1846) and red shrimp (Plesionika martia, A. Milne-Edwards, 1883). J. Anim. Vet. Adv. 8, 183–189.
Orr, D., and Sullivan, D. (2013). The February 2013 assessment of northern shrimp (Pandalus borealis) off Labrador and northeastern Newfoundland. Khalini: DFO.
Papadopoulos, L. S., and Finne, G. (1986). Effect of environmental salinity on sensory characteristics of penaeid shrimp. J. Food Sci. 51, 812–814. doi: 10.1111/j.1365-2621.1986.tb13938.x
Pillet, M., Dupont-Prinet, A., Chabot, D., Tremblay, R., and Audet, C. (2016). Effects of exposure to hypoxia on metabolic pathways in northern shrimp (Pandalus borealis) and Greenland halibut (Reinhardtius hippoglossoides). J. Exp. Mar. Biol. Ecol. 483, 88–96. doi: 10.1016/j.jembe.2016.07.002
Pinheiro, J., Bates, D., DebRoy, S., and Sarkar, D. R Core Team (2018). nlme: Linear and Nonlinear Mixed Effects Models_. R package version 3.
Pörtner, H. O., and Lanning, G. (2009). Oxygen and capacity limited thermal tolerance. Fish Physiol. 27, 143–191. doi: 10.1016/s1546-5098(08)00004-6
Pourang, N., and Amini, G. (2001). Distribution of trace elements in tissues of two shrimp species from Persian Gulf and effects of storage temperature on elements transportation. Water Air Soil Pollut. 129, 229–243.
R Core Team (2018). R: A Language and Environment for Statistical Computing. Vienna: R Foundation for Statistical Computing.
Raymont, J. E. G., Austin, J., and Linford, E. (1967). The biochemical composition of certain oceanic zooplanktonic decapods. Deep Sea Res. Oceanogr. Abstr. 14, 113–115. doi: 10.1016/0011-7471(67)90033-2
Sabine, C. L., Feely, R. A., Gruber, N., Key, R. M., Lee, K., Bullister, J. L., et al. (2004). The oceanic sink for anthropogenic CO2. Science 305, 367–371. doi: 10.1126/science.1097403
Sarower, M. G., Hasanuzzaman, A. F. M., Biswas, B., and Abe, H. (2012). Taste producing components in fish and fisheries products: a review. Int. J. Food Ferment. Technol. 2:113.
Siferd, T. D. (2015). 2015 Assessment of Northern Shrimp (Pandalus borealis) and Striped Shrimp (Pandalus montagui) in the Eastern and Western Assessment Zones (SFAs Nunavut, Nunavik and Davis Strait). Khalini: DFO.
Small, D. P., Calosi, P., Boothroyd, D., Widdicombe, S., and Spicer, J. I. (2015). Stage-specific changes in physiological and life-history responses to elevated temperature and pCO2 during the larval development of the European lobster Homarus gammarus (L.). Physiol. Biochem. Zool. 88, 494–507. doi: 10.1086/682238
Sokal, R. R., and Rohlf, F. J. (1995). Biometry: The Principles and Practice of Statistics in Biological Research. New York, NY: W. H. Freeman, 68–70.
Stortini, C. H., Chabot, D., and Shackell, N. L. (2017). Marine species in ambient low-oxygen regions subject to double jeopardy impacts of climate change. Glob. Change Biol. 23, 2284–2296. doi: 10.1111/gcb.13534
Tabachnick, B. G., and Fidell, L. S. (2006). Using Multivariate Statistics, 5th Edn. Manhattan, NY: Harper & Row.
Tacon, A. G., and Metian, M. (2013). Fish matters: importance of aquatic foods in human nutrition and global food supply. Rev. Fish. Sci. 21, 22–38. doi: 10.1080/10641262.2012.753405
Tate, R. D., Benkendorff, K., Ab Lah, R., and Kelaher, B. P. (2017). Ocean acidification and warming impacts the nutritional properties of the predatory whelk, Dicathais orbita. J. Exp. Mar. Biol. Ecol. 493, 7–13. doi: 10.1016/j.jembe.2017.03.006
Venugopal, V., and Gopakumar, K. (2017). Shellfish: nutritive value, health benefits, and consumer safety. Comp. Rev. Food Sci. Food Saf. 16, 1219–1242. doi: 10.1111/1541-4337.12312
Vlasblom, A. G., Graafsma, S. J., and Verhoeven, J. T. A. (1977). Survival, Osmoregulatory Ability, and Respiration of Idotea Chelipes (Crustacea, Isopoda) from Lake Veere in Different Salinities and Temperatures. Chicago, IL: Delta Institute.
Wienberg, R. (1980). On the food and feeding habits of Pandalus borealis Krøyer 1838. Arch. Fischerei Wiss. 3, 123–137.
World Health Organization [WHO] (1996). Trace Elements in Human Nutrition and Health. Geneva: World Health Organization.
Keywords: ocean warming, ocean acidification, hypoxia, sensory quality, mortality, seafood
Citation: Chemel M, Noisette F, Chabot D, Guscelli E, Leclerc L and Calosi P (2020) Good News — Bad News: Combined Ocean Change Drivers Decrease Survival but Have No Negative Impact on Nutritional Value and Organoleptic Quality of the Northern Shrimp. Front. Mar. Sci. 7:611. doi: 10.3389/fmars.2020.00611
Received: 14 April 2020; Accepted: 02 July 2020;
Published: 31 July 2020.
Edited by:
Christopher Edward Cornwall, Victoria University of Wellington, New ZealandReviewed by:
Marion Pillet, Université de la Rochelle, FranceCeri Lewis, University of Exeter, United Kingdom
Copyright © 2020 Chemel, Noisette, Chabot, Guscelli, Leclerc and Calosi. This is an open-access article distributed under the terms of the Creative Commons Attribution License (CC BY). The use, distribution or reproduction in other forums is permitted, provided the original author(s) and the copyright owner(s) are credited and that the original publication in this journal is cited, in accordance with accepted academic practice. No use, distribution or reproduction is permitted which does not comply with these terms.
*Correspondence: Mathilde Chemel, mathildechemel@yahoo.fr; Piero Calosi, piero_calosi@uqar.ca